Research Topics
Research Overview
The worldwide push to generate electricity from renewable sources has created a critical need to develop improved energy storage and fuel-production strategies. Recent advances in the conversion of solar and wind energy into electrical energy have been increasingly economical, yet without effective methods for storage, it is impossible to integrate these intermittent resources into the commercial sector without compromising reliability. Research in the Matson Group focuses on using a synthetic inorganic chemistry perspective to address current global issues related to Energy Storage and Production.
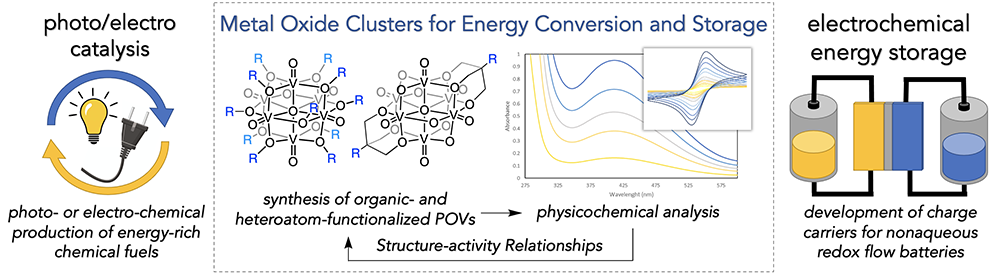
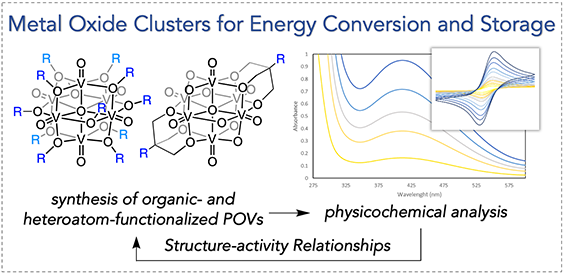
Redox Active Metal-oxide Metalloligands. An attractive solution to the broad challenge of energy storage is to store chemical energy in molecular bonds by converting inert, abundant molecules into energy-rich chemical fuels. As such, the development of new, carbon neutral fuels is a viable approach for sustainable electricity generation. The development of alternative fuels from secure and sustainable resources would decrease our dependence on fossil fuels, and as a result is among the greatest environmental and economic challenge that society faces today. The development of sustainable methods to convert energy-poor substrates to fuels requires the generation of catalysts that can perform a complex series of multi-electron and multi-proton transformations. The Matson Group is investigating new approaches to catalyst design that applies fundamental knowledge from bioinorganic systems and heterogeneous catalyst-support interactions. We have recently discovered a new class of metal-oxide metalloligands capable of participating in cooperative small molecule activation. The main objectives of this research include (i) determining how the electronic properties and activity of a homogeneous, heterometallic catalyst can be influenced by a reducible metal-oxide support and (ii) revealing the role of metal/metalloligand interactions in the cooperative intramolecular electron and proton transport that enables substrate activation. Insights from these investigations will translate broadly into improved designs for homogeneous catalysts targeting the sustainable production of chemical fuels.
Actinide-functionalized metal-oxide clusters. Recent advances in the understanding of the chemistry of 5f-elements have highlighted the potential role of actinide ions in small molecule activation, such as carbon dioxide reduction. However, their chemical behavior in water remains difficult to investigate, as the oxophilicity of fblock elements results in complex chemical interactions of these ions with water. In particular, the extreme moisture-sensitivity of low-valent actinide complexes has led to deleterious side reactions in the presence of trace amounts of water, resulting in the formation of thermodynamically robust An-O bonds. To circumvent these complications, the use of bulky ligand scaffolds that provide steric protection has been developed and met with relative success, though their preparation remains synthetically challenging. The expertise of the Matson group in the synthesis of highly tunable redox active metalloligands places us in a unique position to tackle this challenge. The combination of our POVs clusters with actinides cations constitutes an excellent platform for the investigation of novel An containing species and the study of their electronic properties and potential catalytic activity. This approach also offer access to highly valuable information regarding the electronic structure of atomically precise 3d-5f heterometallic compounds.
Multielectron Charge-carriers for Non-aqueous Redox Flow Batteries. Additionally, the Matson Group is developing earth-abundant, metal-oxide cluster complexes to serve as electrolytes for redox-flow batteries. Redox-flow batteries are among the most promising technologies for grid-scale energy storage. While traditional battery cells rely on internal solid electrodes for energy storage, redox flow batteries use the circulation of two soluble redox couples as electrolytes, rendering these energy storage systems highly modular and functional. In order to make redox flow batteries commercially viable, inexpensive electrolytes that provide dense electrical output must be developed. Our approach to electrolyte design capitalizes on the stability, solubility, and rich redox chemistry of the transition metal-functionalized polyoxovanadate-alkoxide clusters discovered in our laboratory. These heterometallic complexes are generated via single-step, self-assembly pathways from inexpensive, commercial starting materials and undergo multiple highly reversible redox events, making them promising candidates for flow-battery applications.